Introduction
The merging of minds and machines has long been a subject of fascination and exploration in science fiction. However, recent advancements in technology have brought us closer than ever to turning this concept into reality. One of the most promising fields at the forefront of this endeavor is Brain-Computer Interfaces (BCIs). BCIs are revolutionary systems that establish a direct communication pathway between the human brain and external devices, enabling individuals to control machines and interact with technology using their thoughts.
In this comprehensive blog, we will delve into the fascinating world of BCIs, exploring their history, functionality, applications, challenges, and ethical considerations. We will examine the underlying principles behind BCIs, the remarkable technologies that make them possible, and the ways in which they are transforming various domains, from healthcare and assistive technology to gaming and communication. By understanding the capabilities and potential of BCIs, we can gain insights into how they are shaping the future of human-machine interactions.
Furthermore, we will discuss the challenges and ethical considerations surrounding BCIs, such as privacy, informed consent, user autonomy, and equitable access. We will explore the importance of addressing these concerns and implementing responsible practices in the development and use of BCIs. Additionally, we will envision the future prospects of BCIs, envisioning advancements in technology, integration with artificial intelligence and robotics, and the potential impact on fields such as neurorehabilitation, space exploration, and our understanding of consciousness.
Join us on this captivating journey as we explore the Brain-Computer Interface: Merging Minds and Machines. Discover the transformative power of BCIs and gain insights into the possibilities, challenges, and ethical considerations associated with this groundbreaking technology.
Table of Contents
A Brief History of Brain-Computer Interfaces
The concept of connecting the human brain with external devices to enable direct communication and control can be traced back several decades. Let’s take a journey through the key milestones in the history of brain-computer interfaces (BCIs):
1960s-1970s: The Birth of BCIs
The groundwork for BCIs was laid in the late 1960s when researchers began exploring the possibility of using brain signals to control external devices. In 1969, José Delgado, a Spanish-American neuroscientist, conducted pioneering experiments where he implanted electrodes into the brains of animals, allowing him to stimulate and control their behavior. These experiments laid the foundation for future advancements in BCIs.
1970s-1980s: Invasive Techniques and Early Breakthroughs
In the 1970s, researchers made significant progress in recording brain activity and decoding it for basic tasks. One notable achievement during this time was the development of the P300 wave, a specific brain signal related to attention and information processing. Researchers realized that they could use the P300 wave as a marker to detect a person’s intentions, such as selecting an item from a list.
1980s-1990s: Non-invasive Techniques and Electrocorticography (ECoG)
During the 1980s, non-invasive techniques, such as electroencephalography (EEG), gained prominence in BCI research. EEG involves placing electrodes on the scalp to detect electrical activity generated by the brain. Researchers successfully demonstrated the control of simple tasks, such as moving a cursor on a computer screen, using EEG signals.
In the 1990s, another significant development in BCIs was the introduction of electrocorticography (ECoG). ECoG involves placing electrodes directly on the surface of the brain, providing higher-resolution signals compared to EEG. Researchers achieved remarkable control over cursor movement and demonstrated basic communication using ECoG-based BCIs.
2000s-2010s: Advancements in Signal Processing and Machine Learning
The 2000s witnessed substantial advancements in signal processing algorithms and machine learning techniques, which greatly improved the performance and accuracy of BCIs. Researchers developed sophisticated algorithms to decode brain signals and extract meaningful information related to user intent.
One notable breakthrough during this period was the development of the BrainGate system in 2006. BrainGate utilized an array of microelectrodes implanted into the motor cortex of individuals with paralysis, allowing them to control a computer cursor and perform various tasks using their thoughts.
2010s-Present: Non-Invasive BCIs and Neurofeedback
In recent years, non-invasive BCIs have gained significant attention and popularity. Advancements in technology and signal processing have made it possible to extract meaningful information from non-invasive brain signals, such as EEG and functional magnetic resonance imaging (fMRI).
Neurofeedback, a technique that enables individuals to learn to control their brain activity, has emerged as a prominent application of non-invasive BCIs. By providing real-time feedback on their brain states, individuals can learn to modulate their brain activity and improve cognitive performance, relaxation, and attention.
Additionally, there has been a growing interest in consumer-grade BCIs, with companies developing wearable devices that use EEG signals for applications such as meditation assistance, sleep tracking, and brain-controlled gaming.
Related Articles:
The science behind how our brains process emotions and memories,
The science of color and how it affects our mood and perception
How Does a Brain-Computer Interface Work?
A brain-computer interface (BCI) is a system that enables direct communication and control between the human brain and external devices, bypassing traditional means of interaction such as muscles or speech. BCIs rely on various techniques to acquire, process, and interpret brain signals.
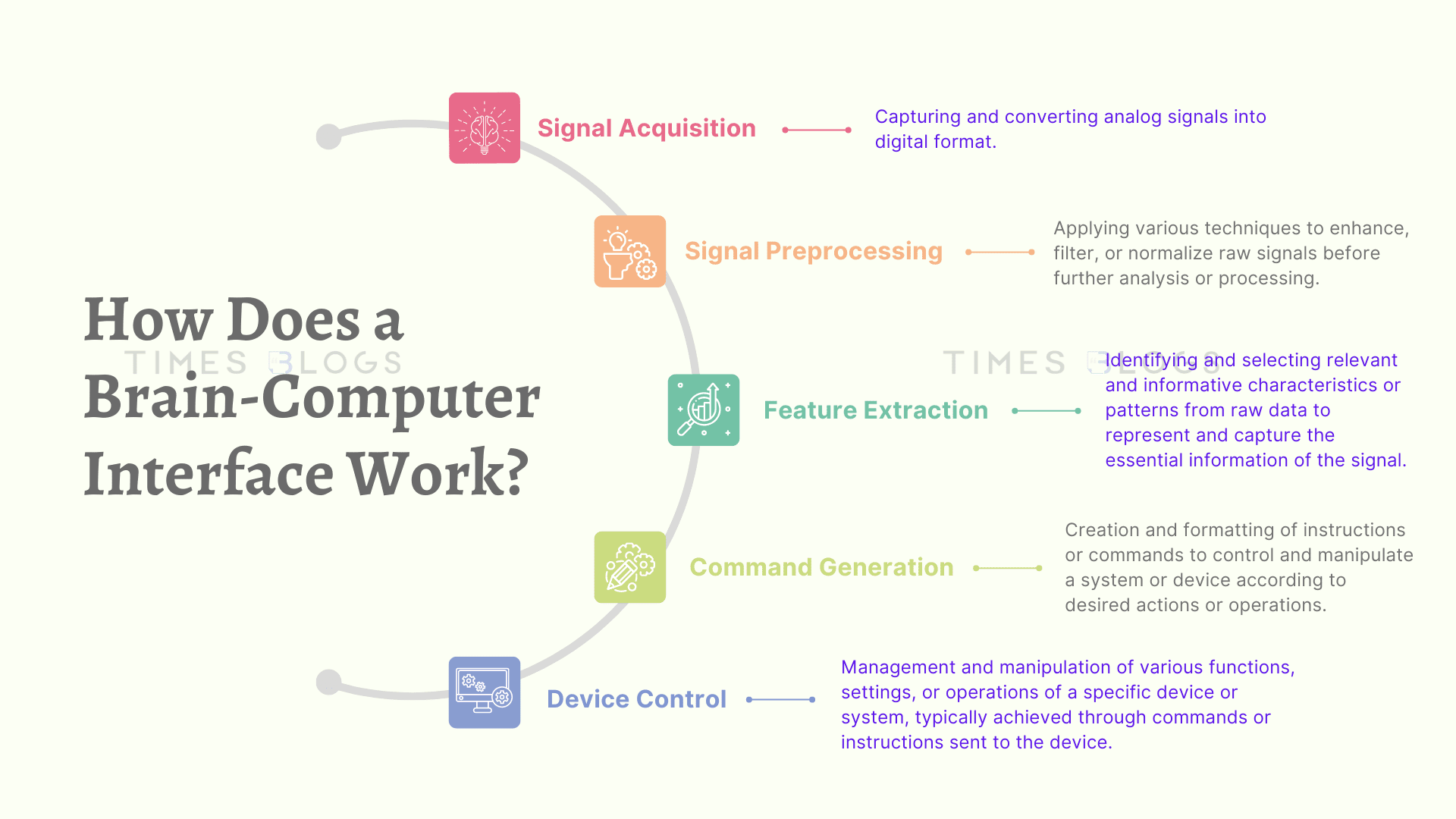
Let’s delve into the key steps involved in the functioning of a BCI:
1. Signal Acquisition:
The first step in a BCI system is to acquire brain signals. BCIs use different techniques to capture these signals, including:
- Electroencephalography (EEG): This non-invasive technique involves placing electrodes on the scalp to measure the electrical activity produced by the brain. EEG is widely used in BCIs due to its accessibility and portability.
- Electrocorticography (ECoG): ECoG involves placing electrodes directly on the surface of the brain, providing higher-resolution signals compared to EEG. It is typically used in invasive BCIs where electrodes are implanted on the brain’s surface.
- Functional Magnetic Resonance Imaging (fMRI): fMRI measures changes in blood flow and oxygenation in the brain, providing information about brain activity. While fMRI offers high spatial resolution, it is less suitable for real-time applications due to its slower acquisition speed.
2. Signal Preprocessing:
Once the brain signals are acquired, they undergo preprocessing to enhance their quality and remove unwanted artifacts. Preprocessing steps may include:
- Filtering: Applying filters to remove noise and unwanted frequencies, focusing on the frequency bands of interest for the specific BCI application.
- Artifact Removal: Identifying and removing artifacts such as eye blinks, muscle activity, and environmental interference to obtain clean brain signals.
- Segmentation: Dividing the continuous signal into smaller segments to analyze specific time intervals relevant to the user’s intentions or mental states.
3. Feature Extraction:
In this step, relevant features are extracted from the preprocessed brain signals. These features capture meaningful patterns or characteristics associated with different mental states or commands. Feature extraction methods can vary depending on the BCI application and the specific brain signal being analyzed. Commonly used techniques include:
- Time-domain features: Extracting statistical measures such as mean, variance, or waveform characteristics from the brain signal.
- Frequency-domain features: Analyzing the power spectrum or extracting frequency band power ratios to capture the distribution of brain activity in different frequency ranges.
- Spatial features: For invasive BCIs, spatial features may involve identifying specific neural population activity patterns related to different tasks or intentions.
4. Command Generation:
Once the relevant features are extracted, they are translated into machine-readable commands that can be used to control external devices or applications. The specific command generation method depends on the BCI design and the intended application. Some approaches include:
- Classification: Using machine learning algorithms, such as support vector machines (SVMs) or artificial neural networks (ANNs), to classify the extracted features into different classes or commands. The user’s intended action or mental state is inferred based on the classification result.
- Regression: Estimating continuous variables or parameters, such as the position of a cursor, based on the extracted features. Regression models can provide precise control in continuous domains.
- Hybrid Approaches: Combining multiple algorithms or strategies to improve the accuracy and robustness of command generation. Hybrid BCIs may utilize a combination of classification, regression, and other techniques to achieve optimal performance.
5. Device Control:
The final step involves using the generated commands to control external devices or applications. BCIs can interface with a wide range of devices, including prosthetic limbs, robotic systems, computer interfaces, or virtual reality environments. The BCI system translates the user’s intentions into commands that control the desired device, allowing for direct interaction through brain signals.
Applications of Brain-Computer Interfaces
Brain-computer interfaces (BCIs) have the potential to revolutionize various domains by enabling direct communication and control between the human brain and external devices. Here are some notable applications of BCIs:
Assistive Technology:
BCIs hold tremendous promise in assisting individuals with disabilities, particularly those with motor impairments. By bypassing the need for traditional muscular control, BCIs can provide a means for people with conditions like spinal cord injuries, amyotrophic lateral sclerosis (ALS), or locked-in syndrome to regain communication and control.
- Neuroprosthetics: BCIs can be used to control prosthetic limbs, allowing individuals with limb loss or limb paralysis to regain mobility and independence. By interpreting the user’s intentions from their brain signals, BCIs enable intuitive and natural control of prosthetic devices.
- Communication Aids: BCIs can provide alternative communication methods for individuals with severe communication disorders. By translating brain signals into text or synthesized speech, BCIs offer a means of expression for those who are unable to communicate verbally.
- Assistive Robotics: BCIs can be integrated with robotic systems, enabling individuals with motor disabilities to control assistive robots for activities such as feeding, grooming, or mobility assistance.
Healthcare and Rehabilitation:
BCIs have applications in healthcare, particularly in neurorehabilitation and cognitive training.
- Stroke Rehabilitation: BCIs can be used to facilitate motor recovery and rehabilitation after a stroke. By providing feedback and facilitating brain plasticity through neurofeedback or brain stimulation, BCIs can help individuals regain motor function and improve their recovery outcomes.
- Pain Management: BCIs offer potential applications in pain management by providing neurofeedback and enabling individuals to modulate their brain activity to reduce pain perception.
- Cognitive Training: BCIs can be utilized for cognitive enhancement and training. By providing real-time feedback on cognitive states such as attention or relaxation, BCIs can assist in improving focus, attentional control, and mental well-being.
Entertainment and Gaming:
BCIs have the potential to revolutionize the gaming and entertainment industry by providing immersive and interactive experiences.
- Brain-Controlled Gaming: BCIs can enable players to control elements of a game using their thoughts, enhancing the level of engagement and interaction. This can range from simple actions like moving objects to more complex tasks that involve strategy and decision-making.
- Virtual Reality (VR): By integrating BCIs with virtual reality systems, users can navigate and interact with virtual environments using their brain signals, creating a more immersive and intuitive VR experience.
Education and Learning:
BCIs can be applied in educational settings to enhance learning experiences and cognitive development.
- Attention and Focus Monitoring: BCIs can measure and provide feedback on a student’s attention and focus levels during learning activities. This information can be used to optimize teaching methods or provide personalized interventions.
- Adaptive Learning: BCIs can be integrated with adaptive learning systems to tailor educational content based on the user’s cognitive states, preferences, and engagement levels. This personalized approach can optimize learning outcomes.
Research and Neuroscience:
BCIs play a crucial role in advancing our understanding of the brain and cognitive processes. They provide a means to study neural activity, cognitive functions, and brain disorders.
- Brain Mapping and Neuroimaging: BCIs can provide valuable data for brain mapping and studying brain networks. Techniques such as functional connectivity analysis can help unravel the complex relationships between different brain regions.
- Cognitive Neuroscience: BCIs enable researchers to investigate cognitive processes, such as attention, memory, and decision-making, by monitoring and analyzing brain signals associated with specific cognitive tasks.
- Brain-Computer Interface Research: BCIs themselves are a subject of active research, with scientists continuously improving signal processing techniques, developing new algorithms, and exploring novel paradigigms for more efficient and accurate BCI systems. Research efforts focus on improving signal acquisition, signal processing algorithms, machine learning techniques, and user experience.
Mental Health and Well-being:
BCIs have potential applications in mental health assessment, monitoring, and interventions.
- Brain-Based Biomarkers: BCIs can aid in the identification and monitoring of biomarkers associated with mental health conditions such as depression, anxiety, or attention-deficit/hyperactivity disorder (ADHD). These biomarkers can assist in diagnosis, treatment planning, and evaluating the effectiveness of interventions.
- Neurofeedback Therapy: BCIs can be used in conjunction with neurofeedback techniques to train individuals to modulate their brain activity and regulate their emotional states. This approach shows promise in treating conditions such as anxiety, post-traumatic stress disorder (PTSD), and attention disorders.
Personalized Computing and User Experience:
BCIs can enhance personalized computing experiences by adapting interfaces and devices to individual users based on their cognitive states and preferences.
- Adaptive Interfaces: BCIs can enable interfaces to dynamically adjust their layout, complexity, or interaction methods based on the user’s cognitive load, attention, or abilities. This adaptive approach enhances usability and accessibility for a wide range of users.
- Brain-Driven User Experience: BCIs can enable devices to proactively anticipate user intentions and preferences, providing a seamless and intuitive user experience. For example, smart home systems could adjust lighting, temperature, or music based on the user’s mental state.
Challenges and Ethical Considerations in Brain-Computer Interfaces (BCIs)
While brain-computer interfaces (BCIs) offer tremendous potential and exciting possibilities, there are several challenges and ethical considerations that need to be addressed to ensure their responsible development and use. Here are some key challenges and ethical considerations associated with BCIs:
1. Privacy and Data Security:
BCIs involve the collection and analysis of highly sensitive brain signals, which raises concerns about privacy and data security. It is essential to protect the confidentiality of individuals’ brain data, as it provides insights into their cognitive states, emotions, and potentially other personal information. Robust security measures, encryption protocols, and strict data handling procedures should be in place to safeguard the privacy and prevent unauthorized access or misuse of brain data.
2. Informed Consent:
Informed consent is crucial when involving human participants in BCI research or applications. Participants should be provided with clear and comprehensive information about the purpose, risks, benefits, and potential implications of participating in BCI studies or using BCI devices. Informed consent should also include the disclosure of any potential side effects, limitations, and the extent of control users will have over their brain signals and data.
3. User Autonomy and Agency:
BCIs have the potential to influence and manipulate individuals’ brain activity and cognitive processes. It is essential to ensure that users have full autonomy and agency over the use and control of their brain signals. Users should have the right to decide when and how their brain signals are used, and they should be able to give or withdraw consent at any time. BCIs should empower users and respect their cognitive privacy and freedom of thought.
4. Bias and Discrimination:
BCIs should be designed and implemented in a way that avoids bias and discrimination. As with any technology, there is a risk of perpetuating existing biases or inequalities. BCIs should be developed with inclusive design principles, ensuring equal access and usability for individuals with diverse backgrounds, abilities, and cognitive profiles. Care should be taken to prevent any unintended biases in signal processing algorithms or user interfaces that could disproportionately affect certain populations.
5. Reliability and Accuracy:
The reliability and accuracy of BCIs are critical factors for their successful adoption and use. BCIs should strive for high performance and minimize errors in interpreting and translating brain signals into commands or actions. Robust signal processing algorithms, calibration procedures, and user training protocols should be developed to ensure consistent and accurate performance. Additionally, transparent reporting of BCI performance metrics and limitations is necessary to set realistic expectations for users and stakeholders.
6. Long-term Effects and Risks:
The long-term effects of using BCIs are still not fully understood. Continuous monitoring and long-term use of BCIs raise questions about the potential impacts on brain health, cognitive function, and psychological well-being. Ethical considerations include conducting long-term studies to evaluate the safety, efficacy, and potential risks associated with BCI use. Additionally, ensuring adequate follow-up and support for individuals using BCIs is crucial to address any potential adverse effects or unintended consequences.
7. Access and Equity:
BCIs should be accessible and affordable to ensure equitable distribution and benefit for all individuals. It is important to bridge the “BCI divide” by addressing technological barriers, reducing costs, and increasing availability for individuals who could benefit from BCIs, particularly those in underserved communities or low-resource settings. Ethical considerations include promoting equal access, addressing socioeconomic disparities, and avoiding the exacerbation of existing inequities in healthcare and technology.
8. Dual-Use and Ethical Governance:
BCIs, like any technology, have the potential for dual-use, where the same technology can be used for beneficial or harmful purposes. Ethical governance frameworks and guidelines are necessary to ensure responsible development, use, and regulation of BCIs. Collaboration between researchers, policymakers, and ethicists is crucial in establishing guidelines, standards, and regulations to address the ethical challenges associated with BCIs. Ethical governance should promote transparency, accountability, and responsible innovation, while also considering the potential societal impacts and implications of BCI technology.
9. Psychological and Cognitive Implications:
BCIs can have psychological and cognitive implications for users. The ability to control or manipulate brain activity may raise ethical concerns related to personal identity, agency, and the blurring of boundaries between the self and technology. It is important to consider the potential psychological effects and ensure that users’ well-being is prioritized in the development and use of BCIs. Ethical considerations include conducting psychological assessments, providing adequate support, and addressing any potential psychological risks or adverse effects associated with BCI use.
10. Social and Ethical Norms:
BCIs have the potential to challenge existing social and ethical norms. They may raise questions about privacy, consent, cognitive enhancement, and the definition of disability. Societal discussions and engagement are necessary to establish consensus on the ethical boundaries, societal norms, and acceptable uses of BCIs. Public dialogue, interdisciplinary collaboration, and involvement of diverse stakeholders are crucial in shaping the ethical framework surrounding BCIs.
Addressing these challenges and ethical considerations requires a multidisciplinary approach, involving researchers, engineers, ethicists, policymakers, and end-users. Collaborative efforts can help establish ethical guidelines, technical standards, and regulatory frameworks to ensure the responsible development, deployment, and use of BCIs. By addressing these challenges and ethical considerations, we can harness the full potential of BCIs while safeguarding individual rights, promoting equitable access, and fostering societal benefits.
Future Prospects of Brain-Computer Interfaces (BCIs)
The field of brain-computer interfaces (BCIs) is rapidly evolving, and the future holds great promise for advancements and novel applications. Here are some future prospects of BCIs:
Improved Performance and Accuracy:
Continued research and technological advancements will likely lead to significant improvements in the performance and accuracy of BCIs. Signal processing algorithms, machine learning techniques, and neuroimaging technologies will become more sophisticated, enabling more precise interpretation and translation of brain signals into commands or actions. This will enhance the usability and reliability of BCIs, making them more efficient and intuitive for users.
Enhanced Non-Invasive Techniques:
Current non-invasive BCIs primarily rely on electroencephalography (EEG) to detect and analyze brain signals. Future developments may bring advancements in non-invasive techniques such as functional near-infrared spectroscopy (fNIRS), magnetoencephalography (MEG), or ultrasound-based methods. These techniques have the potential to offer higher spatial resolution, improved signal quality, and the ability to capture more nuanced brain activity, leading to more robust and accurate BCIs.
Miniaturization and Wearable Devices:
Advancements in hardware technology will lead to the miniaturization of BCI components, making them more compact, lightweight, and portable. This will result in the development of wearable BCIs that can be seamlessly integrated into everyday life. Wearable BCIs will provide greater freedom of movement and enable continuous monitoring and interaction without significant disruption to the user’s daily activities.
Brain-Computer Interface Augmentation:
BCIs may be used to augment human cognitive capabilities beyond their natural limitations. For example, BCIs could enhance memory, attention, or learning abilities by providing real-time feedback and stimulation to optimize brain functioning. This concept of cognitive augmentation has the potential to revolutionize education, training, and cognitive enhancement programs.
Brain-to-Brain Communication:
Research on brain-to-brain communication, also known as brain-to-brain interfacing (BBI), is an emerging area within the BCI field. BBI aims to establish direct communication between the brains of two or more individuals. Although still in its early stages, BBI has shown promising results in laboratory settings. In the future, BBI could enable new forms of communication, collaboration, and even shared experiences between individuals, with potential applications in telepathic communication or cooperative problem-solving.
Neuroprosthetics and Sensory Feedback:
Advancements in neuroprosthetics will enhance the integration of BCIs with artificial limbs and sensory devices. BCIs will enable more natural and intuitive control of prosthetic limbs, allowing users to regain a sense of touch, proprioception, and motor control. Sensory feedback through BCIs will provide users with real-time information about their prosthetic limbs’ interaction with the environment, enhancing their perception and motor skills.
Brain Health Monitoring and Treatment:
BCIs can play a significant role in monitoring brain health and diagnosing neurological disorders. By continuously monitoring brain activity, BCIs can provide early detection of abnormalities or changes in brain patterns, facilitating timely medical interventions. BCIs can also be used for targeted neuromodulation techniques to treat conditions such as epilepsy, Parkinson’s disease, depression, and chronic pain.
Integration with Artificial Intelligence and Robotics:
The integration of BCIs with artificial intelligence (AI) and robotics will open up new possibilities in the field of human-robot interaction. BCIs can enable seamless control and communication between humans and robots, facilitating collaboration in various domains, including healthcare, manufacturing, and assistive robotics. This integration will pave the way for more intelligent, adaptive, and responsive robotic systems.
Ethical, Legal, and Social Implications (ELSI) Research:
As BCIs continue to advance and become more integrated into our lives, it is crucial to conduct robust research on the ethical, legal, and social implications (ELSI) of BCIs. ELSI research will help address concerns related to privacy, data security, informed consent, user autonomy, bias, discrimination, and the impact of BCIs on personal identity and social norms.
Brain-Machine Hybrid Systems:
In the future, we may witness the development of brain-machine hybrid systems, where BCIs are seamlessly integrated with external devices, such as exoskeletons or drones. These systems will enable direct and intuitive control of external devices using the power of the user’s thoughts, expanding the capabilities and reach of human-machine interactions.
Brain-Internet Interfaces:
With the increasing connectivity of devices and the rise of the Internet of Things (IoT), BCIs may eventually be integrated with the internet, creating brain-internet interfaces. This could enable direct communication between the human brain and cloud-based services, providing access to vast amounts of information, AI algorithms, and remote computing capabilities. Brain-internet interfaces could revolutionize fields such as education, research, and entertainment.
Neural Rehabilitation and Brain Plasticity:
BCIs hold great potential for neural rehabilitation and promoting brain plasticity. By providing precise and targeted feedback, BCIs can aid in the recovery of motor functions in individuals with neurological injuries or disorders. They can also be used to promote neural plasticity, facilitating learning and recovery processes in the brain.
Brain-Mapping and Understanding Consciousness:
The use of BCIs in research can contribute to our understanding of the brain and consciousness. BCIs provide a unique opportunity to map and decode brain activity, leading to insights into how different regions of the brain are interconnected and how they give rise to consciousness. This research may shed light on fundamental questions about human cognition, perception, and the nature of consciousness itself.
Brain-Computer Interfaces in Space Exploration:
BCIs could play a significant role in space exploration and long-duration space missions. Monitoring and assessing the cognitive states and mental well-being of astronauts in real-time through BCIs can help optimize their performance, manage stress levels, and detect early signs of cognitive fatigue. BCIs may also enable direct communication between astronauts and spacecraft systems, enhancing efficiency and safety during space missions.
Conclusion
The field of brain-computer interfaces holds immense promise for merging minds and machines, revolutionizing the way we interact with technology and augmenting our cognitive abilities. Through advances in signal acquisition, processing algorithms, and interface design, BCIs are becoming increasingly sophisticated and accessible. However, significant challenges and ethical considerations need to be addressed to ensure the responsible and ethical development and deployment of this technology.
As we venture into a future where BCIs become more integrated into our daily lives, it is crucial to strike a balance between innovation and ethical considerations. Proper regulations, privacy safeguards, and transparent practices are essential to protect the rights and well-being of BCI users. With responsible development, BCIs have the potential to empower individuals with disabilities, enhance human cognitive capabilities, and reshape various fields, from medicine to entertainment.
The merging of minds and machines through brain-computer interfaces represents a remarkable frontier in human-machine interaction. As we continue to explore this exciting field, we must approach it with careful consideration, striving for a harmonious integration that brings about positive advancements while respecting the boundaries of ethics and privacy. By doing so, we can unlock the full potential of BCIs and pave the way for a future where our minds and machines work in harmony for the betterment of humanity.